Comparative Physiology of the Eastern Oyster, Crassostrea virginica and the Asian Oyster, Crassostrea ariakensis
Throughout the past century the Chesapeake Bay population of C. virginica has been in decline due to over-harvesting, habitat destruction, and parasitic diseases such as Perkinsus marinus. However, the morphologically and taxonomically similar Asian oyster, C. ariakensis is more resistant to dermo disease and more resilient to its effects when infected. This led to the consideration of C. ariakensis for introduction to the Chesapeake Bay in order to augment the native population, thereby aiding in ecosystem services and providing an alternative species for the oyster commercial industry. The potential introduction of C. ariakensis to part of the native range of C. virginica led to numerous studies comparing the anatomy and physiological of these two species including some conducted by students in the Paynter Lab.
Aerobic Metabolic Rate
One possible mechanism to explain the elevated growth rate observed in C. ariakensis compared to C. virginica is different basal metabolic rates. We tested this hypothesis with a collaborating researcher at Juniata College by assessing the aerobic metabolic rate of C. virginica and C. ariakensis spat and adults during normal oxygen conditions. We found that adults exhibited the same mass-specific metabolic rate, but C. ariakensis spat consumed more oxygen than C. virginica spat. Therefore, the mechanism for the difference in growth rate is still unknown.
Figure 1. Oysters in airtight respiration chambers in an environmental control room.
For more information on this project please see our publications page. Specifically Harlan 2007 and Lombardi 2012.
Surviving Low Oxygen (hypoxic) and No Oxygen (anoxic) Environments
Hypoxic environments are becoming increasing common and severe both in the Chesapeake Bay and globally. Crassostrea virginica has a well documented ability to withstand hypoxia however little was known about the hypoxic tolerance of C. ariakensis. Further, comparative physiology studies may not only quantify difference in tolerance but may understand mechanisms for hypoxic survival. Therefore, we performed a series of studies on the role of hypoxia and associated environments (like hypercapnia and acidosis) on C. ariakensis and C. virginica.
Comparison of Mortality Times of Crassostrea ariakensis and Crassostrea virginica during Exposure to Anoxic Stress
We exposed oysters (diploid and triploid) to anoxic environments and calculated the average day of death and found that on average triploid C. ariakensis died on day 4 while triploid C. virginica died on day 15. For diploid, C. ariakensis died on day 13 which was significantly earlier than C. virginica which on average died on day 17.
Analysis of Free Amino Acid Pools in the Gill Tissue of Crassostrea ariakensis and Crassostrea virginica under Normoxic and Anoxic conditions
Assessing changes in free amino acids in the gills after anoxic exposure provides insight in to the metabolic pathways used during hypoxia. When oysters were clamped closed inhibiting normal ventilation and the free amino acid levels were analyzed, we found differences in the rate of accumulation of amino acids between species and based on the oxygen tension of the water. During hypoxia, levels of alanine were higher than during normoxia in both species, but they increased more in C. virginica than C. ariakensis. Crassostrea ariakensis aspartate levels were higher than C. virginica levels during normoxia and increase more than C. virginica during hypoxia. Hypoxic levels of glutamate were significantly higher than normoxic levels. Levels of glutamate in C. ariakensis were two times as high as glutamate levels in C. virginica.
Gaping Response, Blood Chemistry and Adductor Muscle Function
Crassostrea virginica has a remarkable ability to withstand low oxygen conditions. Incidentally, C. ariakensis, died earlier than C. virginica during hypoxic exposure in multiple studies. To better understand what physiological traits enable C. virginica to survive during hypoxia, we compared the physiology of these two similar species. Specifically, we tested which species gaped sooner during hypoxia, the role of gaping on ambient water acidification, and the pH of the blood (hemolymph) when gaping was inhibited. These series of studies were performed with the aim of isolating mechanisms associated with hypoxic tolerance and documenting different responses to hypoxia between two closely related species. When adult oysters were exposed to hypoxic water for 24 hours, C. ariakensis gaped more frequently and wider than C. virginica and gaping was associated with acidification of the ambient water. When gaping was restricted by clamping, the longer an oyster was clamped the more acidic the hemolymph became (presumable due to carbon dioxide accumulation) in both species and a more acidic shift was observed in C. ariakensis.
To test if gaping was due to muscle failure from low oxygen and/or high carbon dioxide (and associated low pH), oysters were immersed in hypoxic, hypercapnic, and both hypoxic and hypercapnic environments and adductor muscle contraction strength and speed was assessed. We found that both species contracted with the same strength and gas treatment and time exposed had no effect. However, oysters within the combined hypoxic and hypercapnic environment contracted more slowly than those in other treatments. Therefore, we believe that gaping is not due to muscle failure, but may be performed as a means to release acidic metabolic byproducts from the tissues to prevent acidosis.
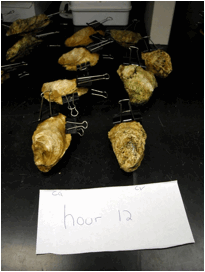
Figure 2. Clamped and emersed C. ariakensis and C. virginica prior to assessing hemolymph pH.
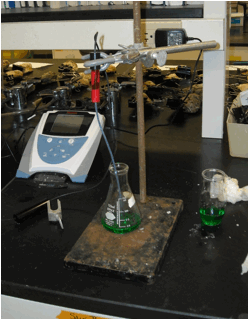
Figure 3. Calibrating the pH meter and micro pH probe.
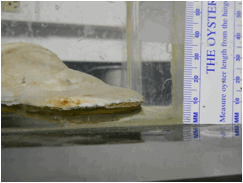
Figure 4. C. ariakensis in a plexiglass chamber filled with hypoxic water gaping.
For more information on this project, please contact us at: paynteroysterlab@gmail.com